Understanding Why Metal Feels Colder Than Wood in Winter
Written on
Chapter 1: The Cold Metal vs. Warm Wood Phenomenon
On a chilly winter morning, touching metal often feels significantly colder than touching wood, despite both being at the same temperature. This sensation is primarily due to the higher thermal conductivity of metals compared to wood. Metals draw heat from your hand at a faster rate, leading to the perception of coldness. However, thermal conductivity is just one factor in this intriguing phenomenon. This article will delve into additional thermal properties that influence our sensory experience.
Let’s conduct a thought experiment.
Imagine you have two slabs, one made of SAE 304 stainless steel (a common type of stainless steel) and the other of teak wood, both at a temperature of 15 °C. Suppose one of your hands is composed of potassium (K) and the other of cobalt (Co), with nearly identical thermal conductivities (k): k_K = 102 W/m·K and k_Co = 100 W/m·K. If both hands are at a normal body temperature of 37 °C, touch the stainless steel slab first with your potassium hand, then with your cobalt hand.
The perceived degree of ‘coldness’ or ‘warmth’ can be measured by the temperature at the interface between your hand and the slab. Table 1 illustrates the interface temperatures (T?) for each combination:

Table 1: Interface Temperatures Analysis
The interface temperatures for the K-teak wood and Co-teak wood combinations are nearly identical. Thus, both hands would perceive the wooden slab as similarly cold. In contrast, the K-SS and Co-SS combinations show a significant difference—the cobalt hand feels the stainless steel slab much warmer compared to the potassium hand. This result is unexpected, as their thermal conductivities are almost equal, suggesting they should extract heat uniformly from the stainless steel slab.
Under steady-state conditions, thermal conductivity solely dictates heat conduction, measuring the rate of heat transfer. A good thermal conductor, like copper, will transfer heat more efficiently than a poor conductor, like wood. However, under transient conditions, both thermal conductivity and thermal diffusivity come into play. Thermal diffusivity (α) is defined as follows:
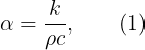
Here, ρ and c represent the density and specific heat capacity of a material, respectively. The product ρc is known as volumetric heat capacity, indicating how quickly a material's temperature changes when heated. Therefore, thermal diffusivity reflects how rapidly a material reaches a steady-state heat transfer after an initial transient period.
Let’s return to our earlier discrepancy. Touching the stainless steel and wood slabs involves transient heat conduction, where both thermal conductivity and diffusivity are crucial. This combination is referred to as thermal effusivity (e):
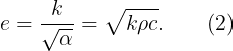
Thermal effusivity measures a material's capacity to exchange heat with its surroundings. If two semi-infinite solids at temperatures T1 and T2 (where T1 ≠ T2) are placed in thermal contact, the interface temperature quickly stabilizes:
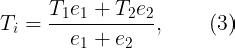
In our scenario, the stainless steel slab, the teak wood slab, and your hand can be treated as semi-infinite solids. When you touch these slabs, the interaction time is brief, allowing this assumption to hold.
The temperatures shown in Table 1 were derived using the equation from earlier. This formula can also predict interface temperatures for different combinations. Table 2 provides these temperatures, comparing the results from Table 1, while Table 3 lists the thermal effusivities of the materials involved.

Table 2: Interface Temperatures for Various Combinations

Table 3: Thermal Effusivities of Materials
In summary, during transient conditions, thermal effusivity influences heat conduction, contributing to our sensations of 'hotness' and 'coldness'.
What happens if you maintain contact with the stainless steel or teak wood slabs for a longer duration? Would this change the interface temperature? Figures 1 and 2 illustrate representative temperature profiles at varying intervals within 5 mm of the interface for the K-SS and K-teak wood combinations.
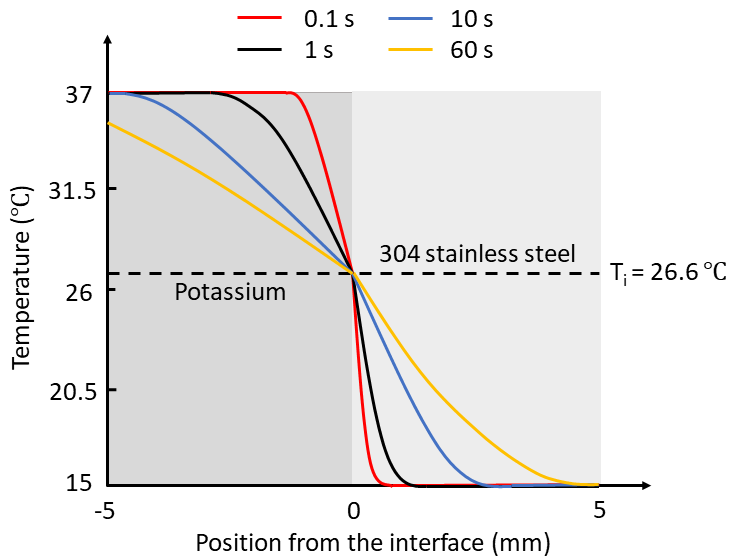
Figure 1: Temperature Profiles for K-SS Combination
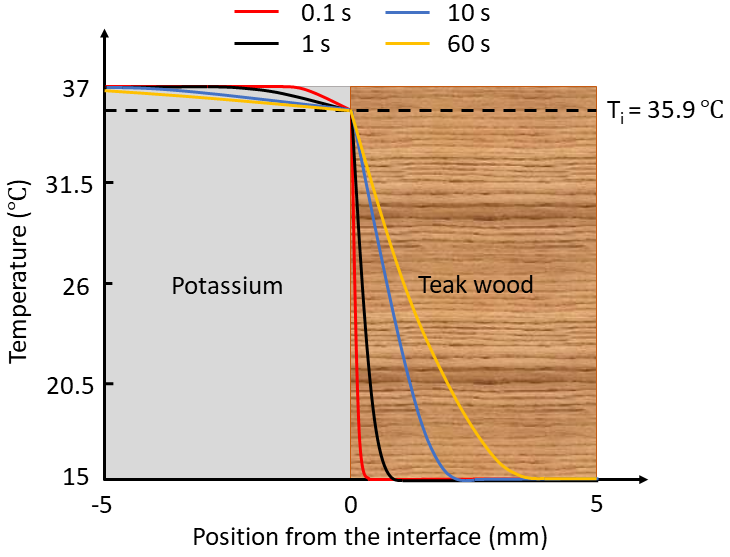
Figure 2: Temperature Profiles for K-teak Wood Combination
Readers are invited to speculate on the temperature profiles for the other combinations. Pay attention to the slopes of the curves!
Food for Thought
For brief periods, temperature profiles within materials change over time, while interface temperatures remain stable. Once steady-state conditions are reached, will the interface temperature differ from the initial temperature?
Consider this scenario: you have a high fever, with a body temperature of 39 °C. A friend, whose temperature is normal, touches your forehead and determines you do not have a fever. What material could their hand be made of? Choose from the following options: (a) glass (b) water (liquid) (c) aluminum (d) diamond (multiple options may apply).
Thank you for reading! Constructive feedback is always appreciated!
Chapter 2: Exploring Thermal Properties
The first video, "Why Does Metal Feel Colder Than Wood? (Explaining the Temperature Perception)," elaborates on the reasons behind our sensory experiences with metal and wood.
The second video, "Why Does Metal Feel Colder Than Wood? - YouTube," provides additional insights into this intriguing phenomenon.